Laser light sources play an important role in light communication systems, but their noise characteristics, which have a significant impact on quality, are of great importance in their operation.
Since the noise characteristics of lasers are a factor in determining the signal-to-noise ratio (SNR) of communications, they are manufactured and designed in accordance with international regulations and standards.
This column describes the different types of laser noise in light communications, as well as their respective standards and evaluation methods.
Table of Contents
- 1. Types of Laser Noise (Relative Intensity Noise and Line width)
- 2. Types of Standards for Relative Intensity Noise
- 3. Evaluation Methods for Relative Intensity Noise
- 4. Types of Standards for Line width
- 5. Evaluation Methods for Line width
- 6. Appendix: Safety Regulations, Safety Measures, and Classification of Lasers
- 7. Conclusion: What are the standards for laser noise in light communications?
- 8. SYCATUS Relative Intensity Noise Measurement System “A0010A”
- 9. SYCATUS Optical Noise Analyzer “A0040A”
1. Types of Laser Noise (Relative Intensity Noise and Line width)
Lasers used in our communication systems are subject to fluctuations in their characteristics as a signal source (noise). Since this affects the communication quality and even the communication capacity, its evaluation and standards are internationally defined. There are two types of laser noise for which evaluation is particularly important.
Relative Intensity Noise (RIN)
Relative Intensity Noise (RIN) refers to the phenomenon of fluctuations in the output intensity of a laser. Fluctuations in optical intensity can adversely affect the SNR of a communication. Management of relative intensity noise is essential for maintaining communication quality and error suppression. In particular, the evaluation of relative intensity noise of laser light is essential to ensure SNR, especially now that the modulation rate of laser light is increasing, modulation schemes are becoming multi-level, and the current of lasers must be reduced to achieve low power consumption.
Line width
Line width refers to the spectral width caused by fluctuations in the frequency (wavelength) of laser light, and is a particularly problematic factor in digital coherent communication systems. If the line width is too wide, accurate demodulation of the communication signal becomes difficult and the error rate increases. For this reason, the line width of laser output is also strictly standardized. Narrower line widths provide higher SNR, which is particularly advantageous for multi-level modulation schemes and long-distance communications.
For reference…
In addition to relative intensity noise and line width, laser safety standards are also defined for lasers used in light communications, and are mainly governed by international standards for laser equipment safety (IEC 60825-1 and IEC 60825-2 “Safety of Laser Products ”*). Each manufacturer is required to design and manufacture laser products in accordance with these regulations.
* The safety regulations and classifications of lasers in IEC 60825-1 and IEC 60825-2 are described in detail in Chapter 6.
2. Types of Standards for Relative Intensity Noise
Standards for relative intensity noise have been established by various international standards to maintain the quality of laser beams. Among them, the standards of the Institute of Electrical and Electronics Engineers (IEEE) are often referred to.
IEEE Standard
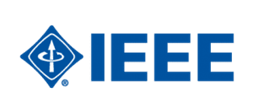
IEEE standards are widely adopted mainly in the field of high-speed data communication and Ethernet communication, and they allow various communication devices to communicate with each other according to a common procedure.
The IEEE 802.3 standard specifies the relative intensity noise of lasers in high-speed communication systems up to 800 Gbps (gigabits per second).
The IEEE 802.3 standard defines the maximum permissible values for each communication method based on the RIN x OMA evaluation item.
3. Evaluation Methods for Relative Intensity Noise
To evaluate relative intensity noise, a high-precision measuring instrument such as a RIN measurement system is used to measure the RIN spectrum and calculate values such as RIN x OMA.
Step 1 Setting up the measurement environment
Adjust the laser output light in its unmodulated state to an appropriate intensity for the measurement using an optical attenuator or similar device. The higher the light intensity, the lower the level of RIN can be measured, but it should not exceed the measurement system's tolerance. When measuring the RIN of a single laser, it is recommended that a low noise laser driver be used. In addition, since RIN depends on the return light to the laser, the return light should be minimized as much as possible, unless the return light is intentionally set as in the case of RIN×OMA.
Step 2 Acquisition of RIN spectrum
Acquire the RIN spectrum by means of a measurement system. Generally, the bandwidth of the spectrum is required to be equal to the modulation rate of the laser light. Since the measurement target is a noise spectrum, it is required to take an average of several dozen times or more.
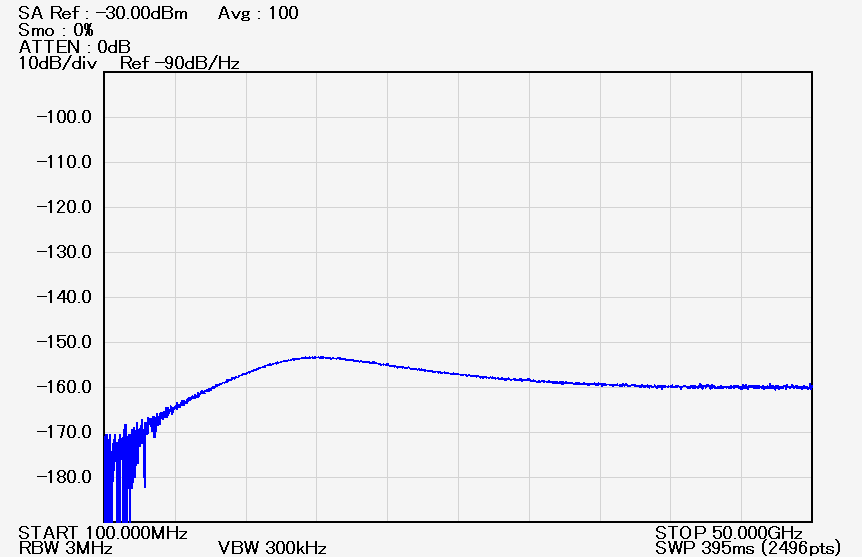
Step 3 Measurement Evaluation
For the measured RIN spectrum, the maximum value is obtained, and the average value is obtained after filtering the spectrum according to the standard.
Step 4 RIN x OMA Evaluation
To obtain the RIN x OMA of IEEE802.3, it is also necessary to measure the power during modulation. With the laser modulated as specified, perform Step 1 through Step 3 above to calculate the value of RINxOMA based on the calculation formula defined in IEEE802.3.
4. Types of Standards for Line width
Standards for line width also have a significant impact on the signal stability of light communications, and many international standards have established standards for line width. In particular, the aforementioned IEEE, OIF (Optical Internetworking Forum), and ITU-T (International Telecommunication Union - Telecommunication Standardization Sector) standards are widely adopted as standards for line width. The line widths of laser light sources are evaluated according to the standards specified by each standard.
IEEE Standard
IEEE defines standards for laser line width, especially for digital coherent systems, which must be lower than the specified value. 802.3ct and 802.3cw use the white noise line width as an evaluation index. For the definition of line width, ITU-T G.698.2 is referenced. To determine the white noise line width, measurement of the optical frequency noise spectrum of the laser is essential. It is impossible to obtain the white noise line width using conventional delayed interferometer line width measurements.
ITU-T standard
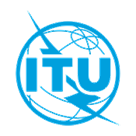
The ITU-T standard G698.2 specifies the line width of lasers for digital coherent systems. Here, as in IEEE, the evaluation of white noise line width is required.
OIF Standard

OIF (Optical Internetworking Forum) is a non-profit organization that develops standards for light communication technology. As a standardization of interconnections in light communications, OIF provides line width standards for lasers, especially as they relate to digital coherent communications between data centers. The line width requirements are expressed as a mask for the optical frequency noise spectrum.
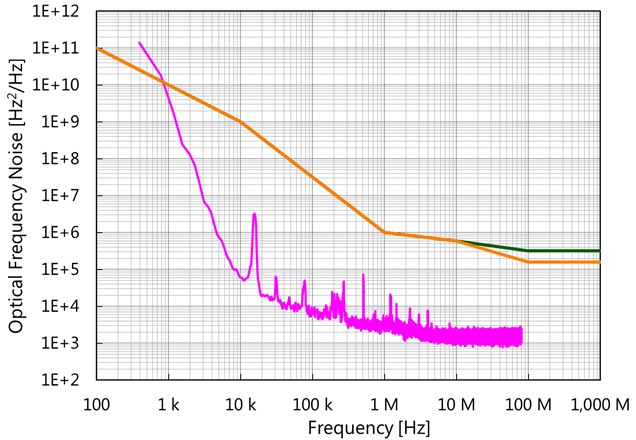
OpenZR+ Standard
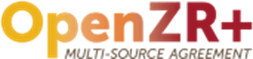
OpenZR+ is a standard specifically for data center-to-data center communications, covering digital coherent light communication technologies from 100G to 400G. Like OIF, OpenZR+ specifies line width characteristics as a mask for the frequency noise spectrum.
5. Evaluation Methods for Line width
Select an appropriate instrument to measure the line width of the laser. Detailed line width measurement, such as white noise line width, is not possible with ordinary optical spectrum analyzers or delay interferometers. An optical noise analyzer or similar instrument capable of evaluating the optical frequency noise spectrum is used.
Step 1 Setting up the measurement environment
Set the laser to the unmodulated state and use an optical attenuator or similar device to adjust the intensity to an appropriate level for the measurement. The higher the optical intensity, the lower level of line width can be measured, but it should not exceed the measurement system's tolerance. When measuring the line width of a single laser, it is recommended to use a low-noise laser driver. Also, since line width is very sensitive to the return light to the laser, an optical isolator should be used when coupling the laser light to the optical fiber.
Step 2 Obtaining the optical frequency noise spectrum
Acquire the optical frequency noise spectrum using an optical noise analyzer or the like. Since the measurement target is the noise spectrum, it is required to take the average of several tens of times or more.
Step 3 Measurement Evaluation
The OIF and OpenZR+ standards need to verify that the optical frequency noise spectrum is below the required mask. In the IEEE and ITU-T standards, the level (unit: Hz2/Hz) of the part of the optical frequency noise spectrum that is flat in the high frequency region (usually 100 kHz or more) on the horizontal axis is extracted, and the value obtained by multiplying that level by π is evaluated. Measurement of the optical frequency noise spectrum is mandatory for all standards.
6. Appendix: Safety Regulations, Safety Measures, and Classification of Lasers
IEC 60825-1 and IEC 60825-2 are IEC standards that specify the safety of laser products.
In order to use lasers safely, it is important to follow the classifications and safety standards according to their power levels and conditions of use.
Class 1 or Class 1M of the following classifications generally applies to laser light sources used for light communications.
Class 1 | Wavelength Range: Covers all wavelengths within safe emission limits. Intensity: Extremely low; does not exceed the Accessible Emission Limit (AEL) for Class 1. Safety: Safe under all conditions of use, including long-term exposure. Often enclosed or inherently safe systems, such as laser printers or fiber optics. |
Class 1M | Wavelength Range: Typically in the infrared (IR) or visible spectrum. Intensity: Low enough to be safe under unaided viewing but may exceed safe limits when viewed with optical aids like binoculars. Safety: Safe for the naked eye; precautions required for magnified viewing. |
Class 2 | Wavelength Range: Restricted to visible light (400–700 nm). Intensity: Limited to 1 milliwatt; safe due to natural aversion responses like blinking or turning away. Safety: Brief exposure is safe; intentional staring or bypassing aversion responses can pose risks. |
Class 2M | Wavelength Range: Visible light (400–700 nm). Intensity: Similar to Class 2; safe limits for unaided viewing but may pose risks when magnified. Safety: Avoid using magnifying optics; otherwise, safety is similar to Class 2. |
Class 3R | Wavelength Range: Covers visible, IR, and ultraviolet (UV) regions. Intensity: Power levels up to 5 milliwatts. Safety: Low risk for accidental exposure, but direct viewing of the beam or prolonged exposure can be hazardous. |
Class 3B | Wavelength Range: Includes visible, IR, and UV regions. Intensity: Power between 5 milliwatts and 500 milliwatts; can cause immediate eye injury. Safety: Requires protective eyewear and controlled environments; diffuse reflections are generally safe. |
Class 4 | Wavelength Range: Applicable to all wavelengths. Intensity: Exceeds 500 milliwatts; high-intensity beams capable of burning skin, damaging eyes, or igniting materials. Safety: Strict safety measures required; exposure to direct, reflected, or scattered beams is hazardous. |
Class 1 lasers are lasers with power levels that are considered harmless and can be used in a safe environment. Class 1 lasers do not require special warning labels, but manufacturers carefully define the environment and methods of use to ensure that users do not accidentally handle high power.
Class 4 lasers are the highest power and most hazardous classification. Class 4 lasers are hazardous to the eyes and skin due to direct or reflected light. For this reason, warning labels and safety measures are mandatory when working with Class 4 lasers, and there are legal provisions for the wearing and use of appropriate protective equipment.
IEEE 802.3 requires that laser output be designed so that it does not exceed certain intensity levels, and also specifies safety labels and warning signs. Lasers fall under class 1 or 1M, which requires users to take appropriate safety measures.
Reference:
【レーザー製品の安全基準】レーザークラスの分類と安全対策、関連する法令等について解説|株式会社日本レーザー ([Safety Standards for Laser Products] Classification of laser classes, safety measures, and related laws and regulation)
レーザーの安全規格|株式会社大興製作所 (Safety Standards for Lasers)
7. Conclusion: What are the standards for laser noise in light communications?
Laser noise in light communications systems and safety regulations are intended to improve the quality of communications and ensure the safety of users. Standards for relative intensity noise and line width of lasers are established based on international standards. In addition, classifications and warning labels require strict safety measures for handling laser beams. As light communications technology evolves further in the future, noise and safety standards for lasers will be reviewed as appropriate and thoroughly enforced.
8. SYCATUS Relative Intensity Noise Measurement System “A0010A”
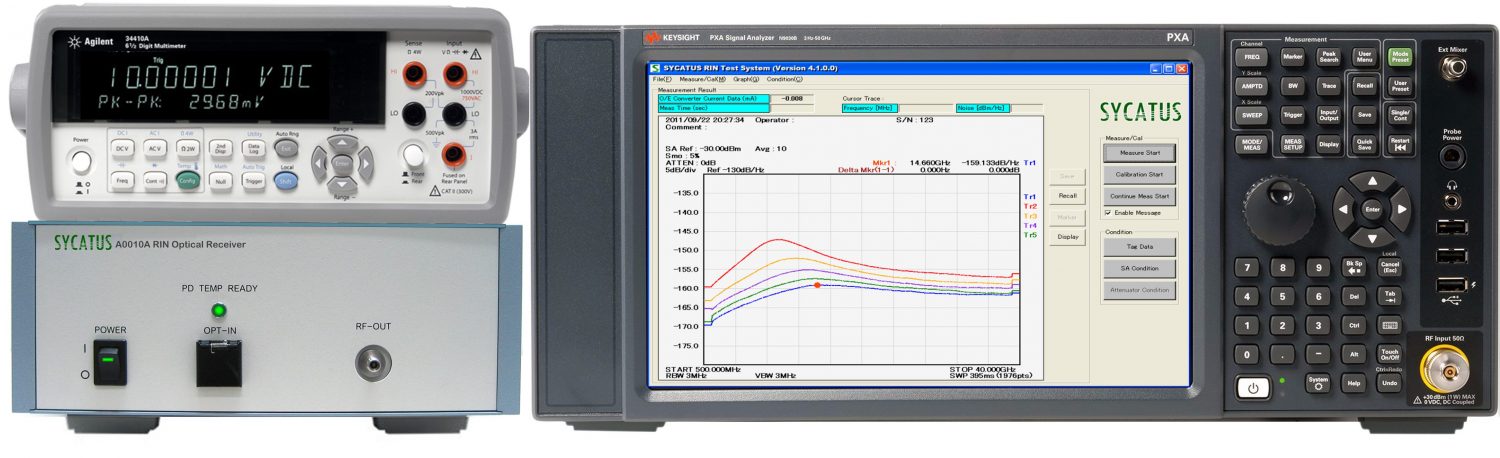
The A0010A RIN measurement system from SYCATUS uses a high-sensitivity, low-noise optical receiver optimally designed for relative intensity noise measurements and a high-performance X-Series signal analyzer from Keysight Technologies, Inc.
The world's widest measurement bandwidth of 50 GHz is used to evaluate the spectrum of relative intensity noise.
9. SYCATUS Optical Noise Analyzer “A0040A”
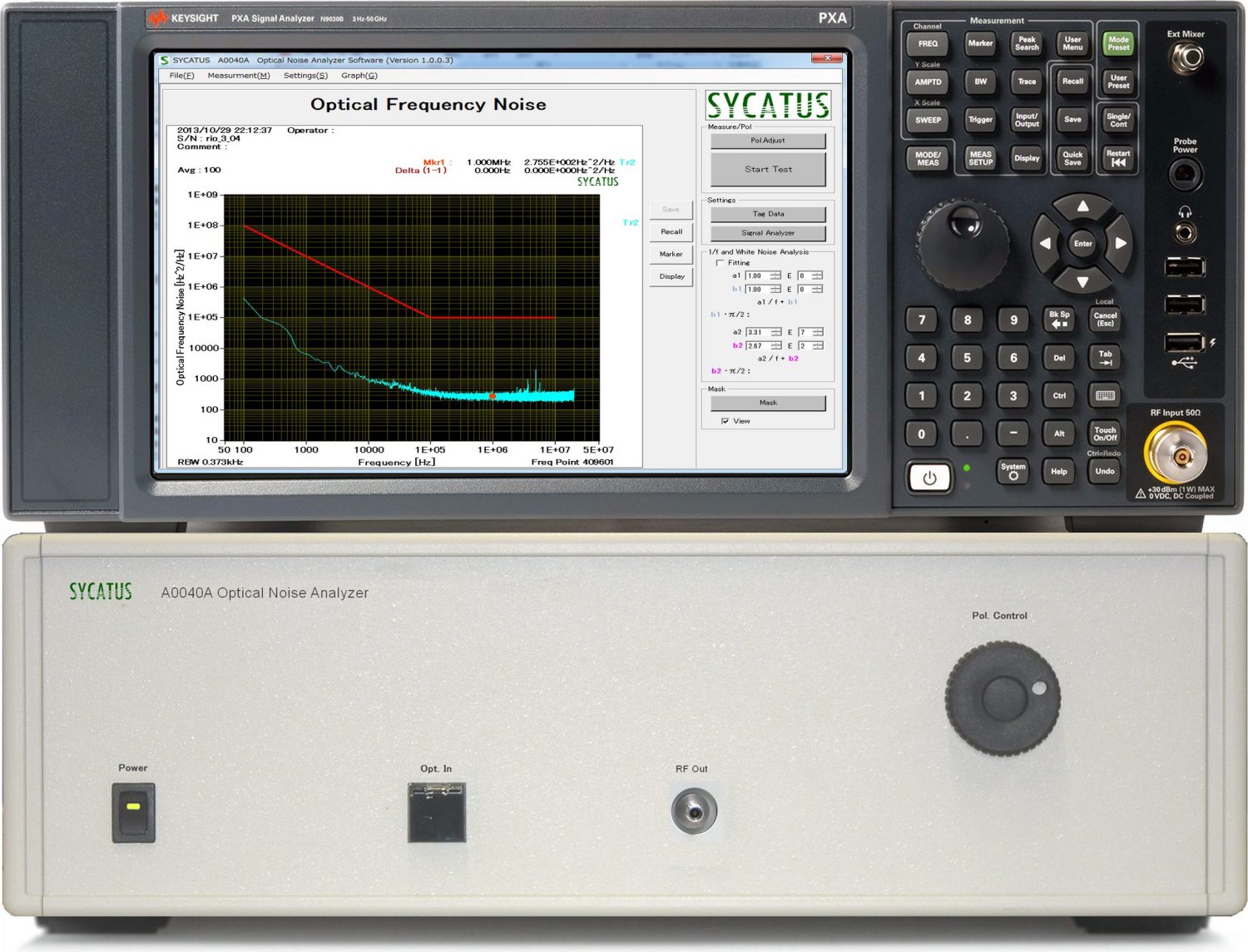
The A0040A optical noise measurement system from SYCATUS is designed to evaluate the line width of a laser as an optical frequency noise spectrum.
It covers a wide wavelength range from O-band to L-band. It provides high repeatability over a wide optical power range of -7 dBm to +3 dBm. Narrow line width measurements down to 0.002 Hz (at 1 MHz abscissa frequency) are possible.
In combination with an electrical signal analyzer, the line width can be easily measured.
Feel free to contact us.
As a pioneer in measurement in the field of light communications and optical sensing, SYCATUS has been providing integrated hardware and software systems for measurement for over 20 years.
We will continue to provide innovative light measurement technologies developed on the basis of expertise, uniqueness, and accuracy to the world.
We also provide expert advice on a wide range of light measurement questions and concerns regarding the installation of system equipment.
Our experts are available to help you solve your light measurement problems.